Factors Affecting Red Wine Colour & Stability
Most winemakers in all regions of Australia seek to present wines to market that have pigmentation that is appropriate for the grape varietal, wine style and consumer acceptability, since a wine’s visual appearance is known to be a strong determinant of the latter (Mercurio et al, 2010). This can present a challenge in some regions where cropping or disease loads may be consistently higher, and various oenological products and winemaking strategies are used to attempt to ameliorate these issues. This can often take the form of one product that is marketed to specifically solve such problems, but the reality that red wine colour management is best tackled through a holistic approach, since there are many contributing factors to red wine colour and stability. This article seeks to canvas some of these strategies.
Phenolic potential
Any grape has a certain intrinsic maximum tannin and pigmentation (together here termed “phenolic”) potential that can be extracted. Achieving complete extraction of these components is impossible, and may not even yield a desirable wine style even if it could be achieved, so for the purposes of this article we shall consider 100% extraction to be in reference to that level of extraction desired for a given grape/wine. Initial phenolic (Bowyer, 2002) potential is determined by several factors, such as varietal, level of fruit ripeness, the physical condition of the fruit during growth and during harvesting, and post-harvest handling. Once at the winery, phenolic potential is then divided into two main areas: extraction and preservation.
The various components contributing to red wine colour are not all extracted at the same rate (Figure 1, Ribéreau-Gayon et al, 2004), however, and this has ramifications in terms of overall wine production management. For example, anthocyanins are relatively aqueous-soluble, and so are easily extracted early in the winemaking process, and this can be further enhanced by cold soaking and/or enzymatic treatment. The time required for cold soaking, however, can be prohibitive in itself in process terms where time is finite and of the essence. Tannins, which can be useful in stabilising anthocyanins, are typically more ethanol-soluble, and so are not extracted until later in the fermentation when some alcohol has been produced, and so in the early part of fermentation we have abundant anthocyanin but insufficient tannin available to chemically stabilise it. Likewise, polysaccharides, which also have a role to play in colour management, come late to the party, in concert with ethanol formation.
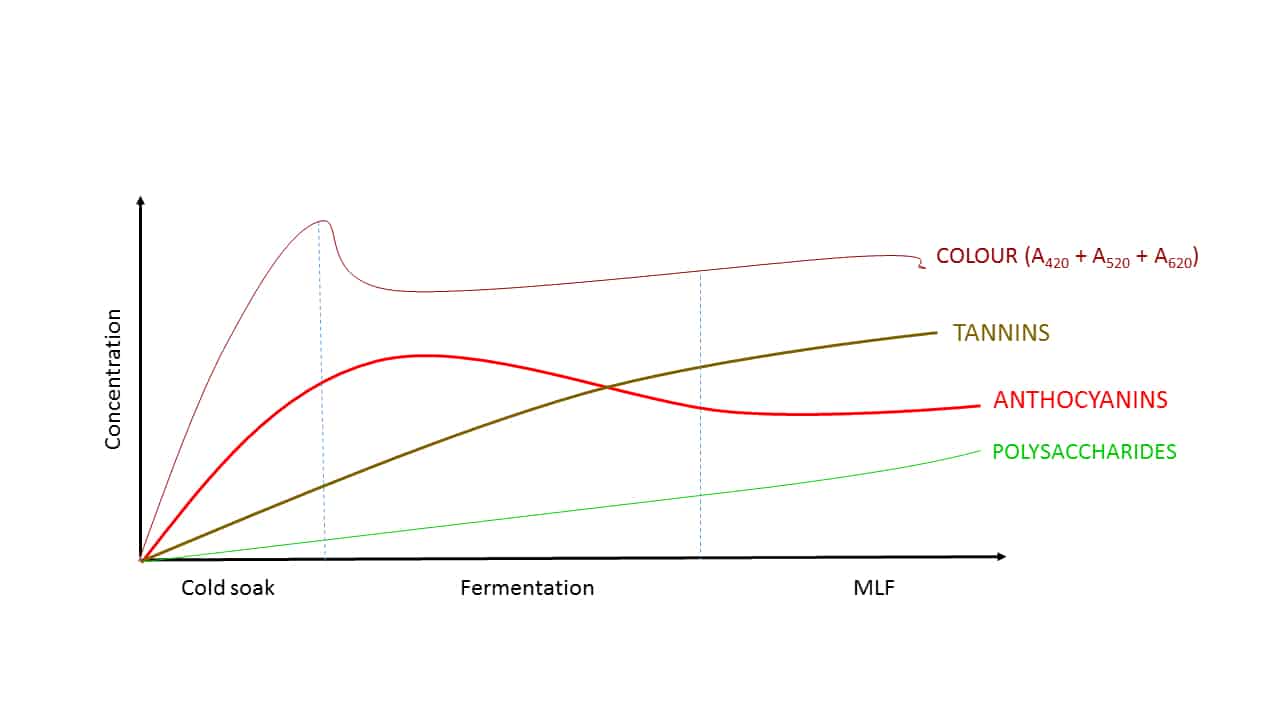
Figure 1. The approximate relative extraction rates of various components that contribute to overall red wine colour. It is for these reasons (and others) that red wine colour management is best approached by considering all contributing factors, not simply individual components.
Enzymes
Since phenolic components reside in the grape cells, and these cells are broken down by enzymes of the correct composition, enzymes inherently play a role in phenolic extraction in wine production (Bowyer et al, 2010). Of particular relevance to extracting the maximum amount of phenolic material, and retaining it, are the activity and purity of the enzyme product used. Purified enzymes typically have greater activity and the absence of unwanted enzymatic side activities. The presence of anthocyanase is particularly undesirable when wine colour and pigment levels are of great importance, as it cleaves the glucose moiety from anthocyanins, reducing molecular and thus colour stability. For example, Oenozym Crush Red is a highly active, fully purified red maceration enzyme. It has typically 2-3 times the activity of a standard winemaking enzyme, and is purified of both cinnamyl esterase (which produces Brettanomyces substrates) and anthocyanase. When compared with a basic non-purified red maceration enzyme (Figure 2), significantly higher colour levels in the final wine are typically observed.
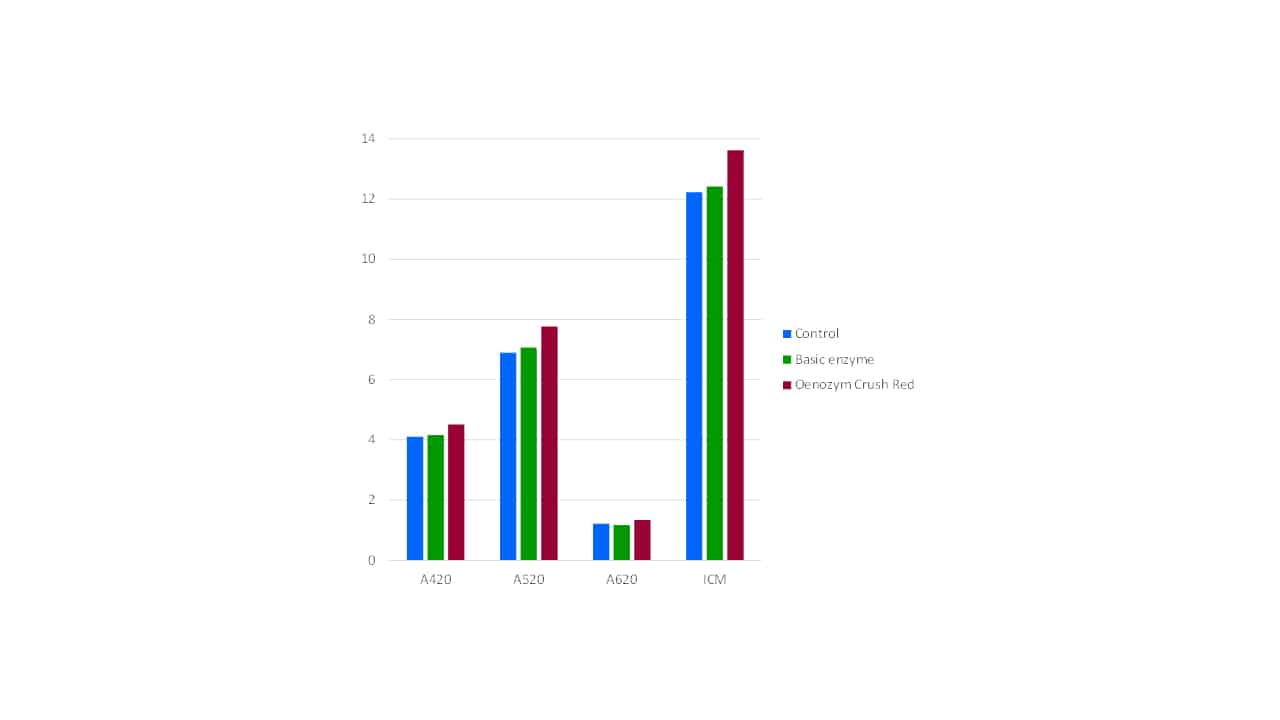
Figure 2. A comparison of final wine colour parameters for control (no enzyme), basic enzyme and purified enzyme treatments.
Yeast choice
Even something as simple as yeast choice for the fermentation is not as straightforward as it seems when considering red wine colour. Some yeasts are known to influence wine tannin levels (Holt et al, 2013), presumably through interactions with proteins on the cell wall outer. In a similar manner, some yeast strains adsorb more pigmentation in the lees than others (Morata et al, 2003), leading to different colour levels in the final wine. This characteristic is given particular consideration in strain selection during hybrid yeast evaluation (Bowyer et al, 2017), especially when the strain in question is being marketed towards lower pigmentation varietals such as Pinot noir. Fermentation kinetics of the yeast strain are important too, since a faster-fermenting yeast necessarily results in reduced skin contact time if the fermentation is pressed early or when sugar depletion is complete. Yeast polysaccharide production also plays a role in wine pigmentation levels (Escot et al, 2001), and certain yeasts are known producers of polysaccharide. Additionally, some yeast-derived fermentation products are specifically formulated to deliver elevated polysaccharide levels to the fermenting must (see Figure 7).
Excellence XR is an interesting yeast strain in this context, in that it is a known polysaccharide producer, and this characteristic can have a significant impact on the colour and phenolic perception of wines fermented with it. Figure 3 shows two wines made from Adelaide Hills Cabernet in the 2019 vintage, produced insofar as possible in a commercial facility under the same conditions, with the only significant variable being the yeast strain used. The wines are shown approximately 8 months after vinification. Basic chemical parameters for the two wines are provided in Table 1. This case represents an extreme example of colour variation in wine by yeast strain, yet Excellence XR almost always delivers superior colour density in such comparisons.
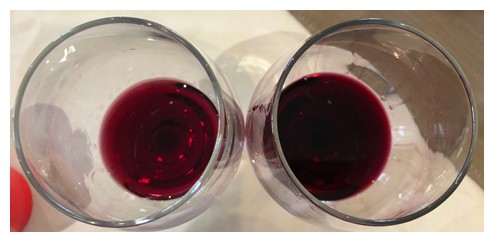
Figure 3. Two wines, identical in all production aspects as far as possible, excepting for the yeast strain used in fermentation. Excellence XR was used in the sample at right.
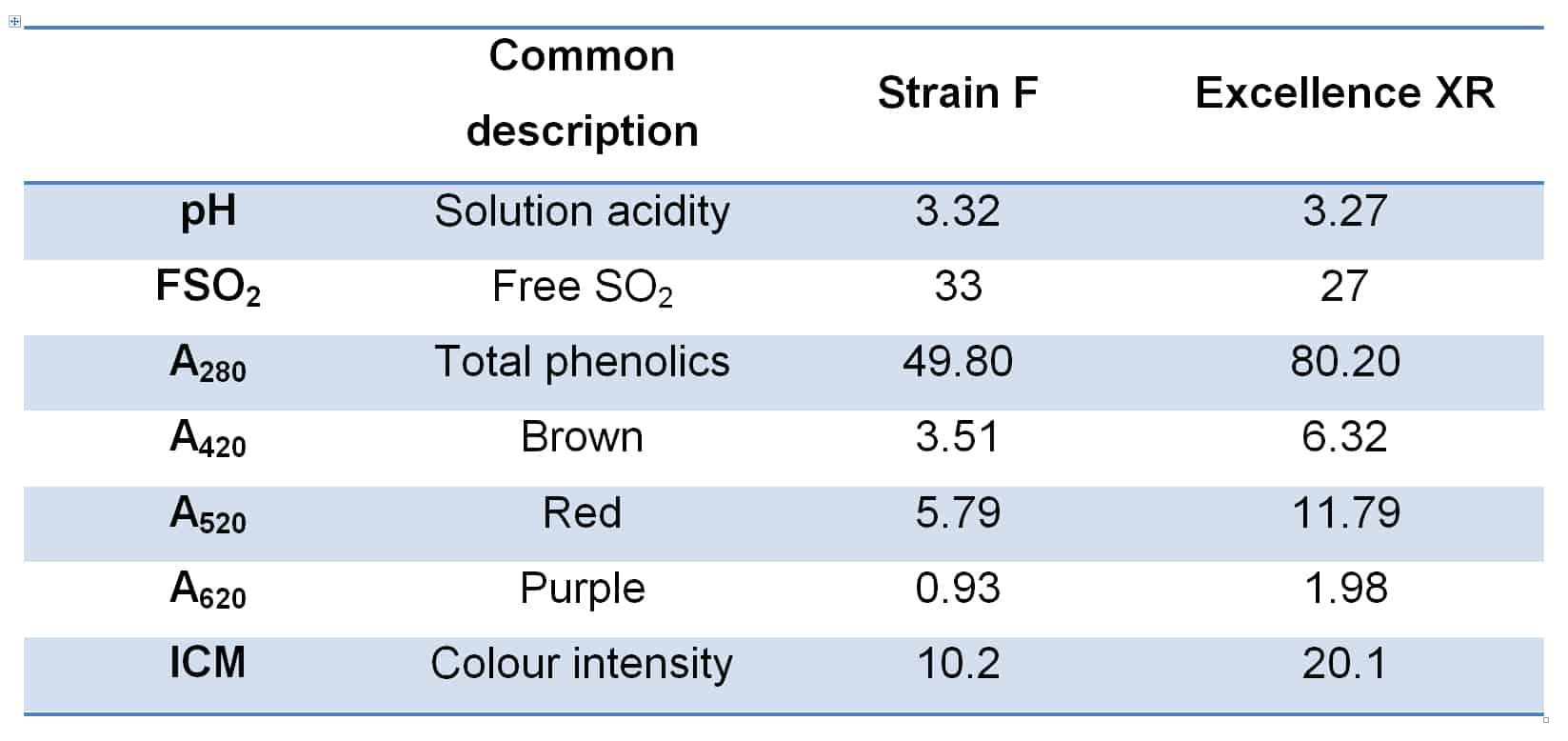
Table 1. Basic chemical parameters for the two wines shown in Figure 2.
Fining tannin
Any reduction of the wine phenolic potential will have a negative impact on red wine colour, hence the preservation of indigenous phenolic material is an important consideration when seeking to maximise red wine colour. When red grapes are crushed and fermentation occurs, components that are kept separate within the grape structure are forcibly mixed, such as grape proteins and grape tannins. This results in a simple fining action, since proteins and tannins aggregate through hydrophobic reactions, and can then flocculate and precipitate, hence some indigenous phenolic components are typically lost. Since grape tannins are not extracted greatly until the fermentation has progressed (Figure 1), a window of opportunity exists for the winemaker to remove or reduce the impact of indigenous grape proteins on final phenolic levels by introducing a “fining” or sacrificial tannin during the aqueous phase of the winemaking process. Caution must be exercised, however, to ensure that any added enzyme has first had a chance to perform its function, since enzymes are simply functional proteins, and so can also interact with added and/or indigenous tannin.
A key characteristic for such a fining tannin is thus protein binding efficiency, and this can be easily measured using a model enzyme (viz., protein) such as laccase, which is the principal phenolic degradation enzyme expressed by Botrytis cinerea. As can be seen in Figure 4, in a wine with a laccase activity of 3 U/mL, early addition of Pro Tanin R @ 100 ppm results in incomplete protein binding (remaining laccase activity of 1 U/mL), whereas increasing the dosage to 200 ppm eliminates the enzyme (0 U/mL), thus inhibiting colour loss and preserving the wine’s phenolic potential. The effectiveness of Pro Tanin R at protein binding is higher in must, since hydrophobic forces are stronger in must than wine. It should also be noted that since Pro Tanin R itself is pigmented, any addition to must or wine will result in higher colour intensity.
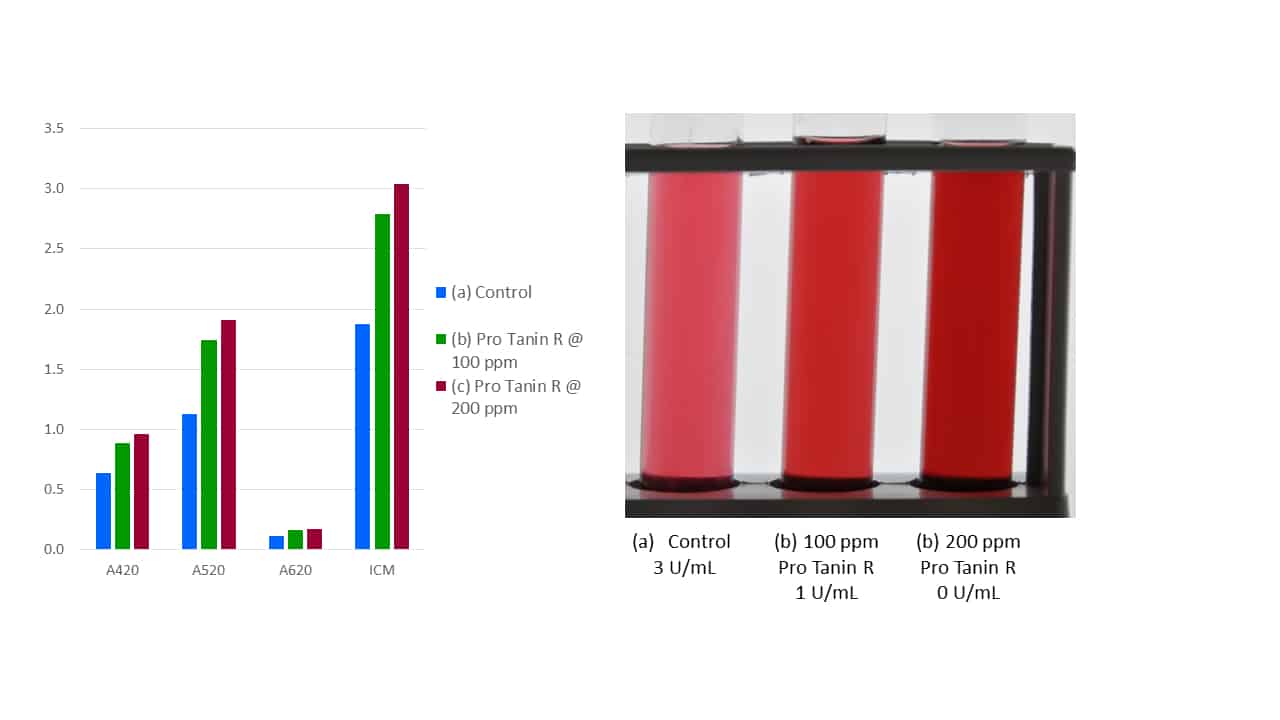
Figure 4. The protein binding efficiency of Pro Tanin R, as indicated by laccase binding in wine.
Colour stabilising tannin
Whilst some tannins are formulated for protein binding, others are formulated for different purposes. Some tannins are rich in grape-derived material, like Tan’Excellence, which aims to elevate and maintain wine redox potential. Other tannins are formulated for colour binding (fixing), and typically contain elevated levels of catechin, a colourless flavan-3-ol that is very efficient at reacting with anthocyanin due to its phloroglucinol molecular core (Bowyer, 2009) to form a colour-stable dimer, which can in turn be incorporated into larger structures. A similar reaction can also occur via ethanal bridging, as depicted in Figure 5.
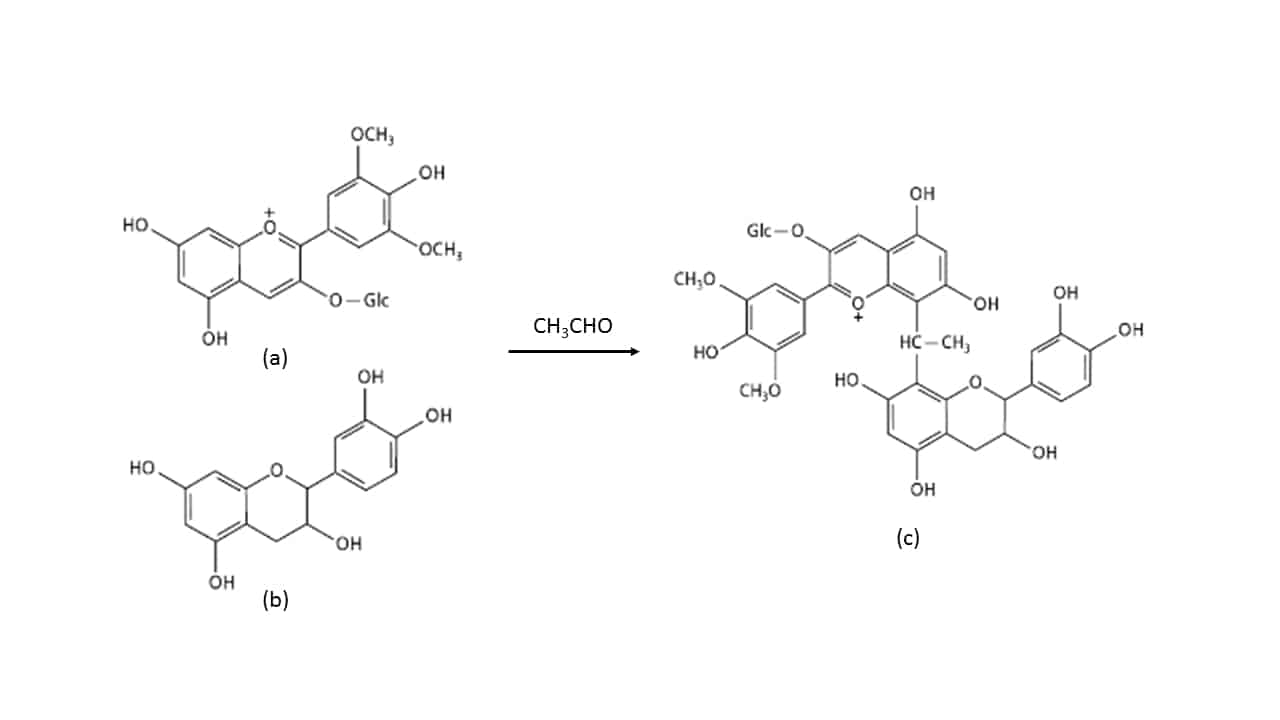
Figure 5. Ethanal (CH3CHO) bridging between an anthocyanin (a, Malvidin-3-glucoside as depicted) and catechin (b; stereochemistry not shown) to form a more stable pigmented product (c; stereochemistry not shown).
Ethanal, as the precursor to ethanol in fermentation, is present in high levels in a fermenting must, and as a highly reactive molecule it can be used to experimentally assess the relative reactivities of various tannin formulations in terms of their colour fixing abilities. Figure 6 shows the elevation in NTU over time for different grape and commercial tannin solutions when an excess of ethanal is introduced. The increases in NTU are correlated anecdotally with the formation of larger molecular species, and thus are a de facto measure of tannin reactivity. Although this experiment does not directly model anthocyanin binding, it is an effective indicator of the capacity of each tannin blend to do so.
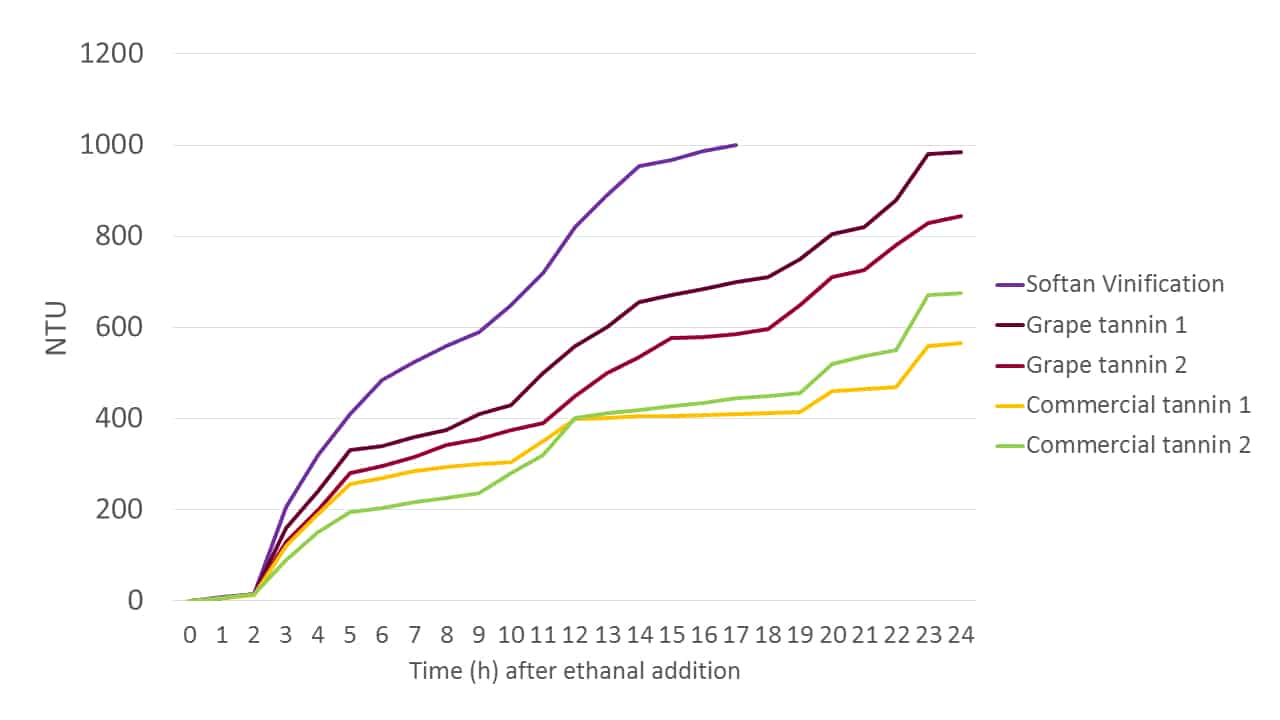
Figure 6. The elevation of NTU in tannin solutions over time in the presence of an excess of ethanal as a de facto indicator of tannin-anthocyanin binding reactivity.
Softan Vinification is evidently rich in catechin, as indicated by its rapid reaction with ethanal. Interestingly, it is not simply a tannin, but is comprised of a blend of catechin-enriched tannin and polysaccharide. Further, these components are not simply combined in a dry state, but rather are blended as solutions and then dehydrated to form an active, “coated” tannin. In this way the chemical reactivity of the tannin is maintained, but the palate impact of the tannin is “softened” through colloidal tannin-polysaccharide hydrophobic associations, as the product name implies. Grape-derived tannins are also shown to be effective at anthocyanin binding, although their higher cost of production requires that commercial tannins containing grape-derived components are typically more expensive.
Maturation tannin
The main role of a maturation tannin is the maintenance of the wine’s redox potential, which can be deficient under some circumstances. Since tannins of various forms are capable of functioning as antioxidants, they can deplete through reaction with oxygen during wine production and maturation. Hydrolysable (oak-derived) tannins are excellent in this role, whereas condensed (grape-derived) tannins are superior at binding anthocyanin. As with any tannin addition, earlier addition typically leads to better palate integration. Many maturation tannin blends are available commercially. Tan’Excellence, for example, is formulated from grape and oak tannin to mimic a red wine’s indigenous tannin profile (assuming some level of low-medium toasted oak exposure), so it supports wine redox potential, elevates the overall tannin profile and can also stabilise colour (see Figure 7).
Comparative treatments
Considering those aspects of colour extraction and preservation previously mentioned, there are other treatments that are used in an attempt at retaining wine colour. For example, additives rich in yeast-derived polysaccharide have already been noted, such as Natur’Soft, and oak is often introduced to fermentations or during maturation in an attempt to enhance colour (in addition to adding oak characters). While oak can certainly introduce tannin to a wine, thereby maintaining its redox potential, the ability of these components is essentially limited to providing components for copigmentation rather than colour fixation. Some aldehydes derived from oak can act as bridging compounds in a similar manner to ethanal, but their concentrations are dependent upon toast levels and are typically low, and oxygen also plays a key role (Bowyer and McCord, 2007).
When various treatments are directly compared for their colour stabilising effectiveness (Figure 7), both in the immediate and longer terms, their relative initial and continuing capacities are revealed. It should be remembered that tannins and oak compounds are typically somewhat pigmented, and so intrinsically contribute to overall colour intensity, whilst polysaccharides are typically colourless. The comparison was not definitive, and addition rates were not uniform, rather being what the wines might see typically in commercial application, in an attempt to replicate a real-world expectation. It can be seen that the impacts of these products on both colour and colour stability vary considerably.
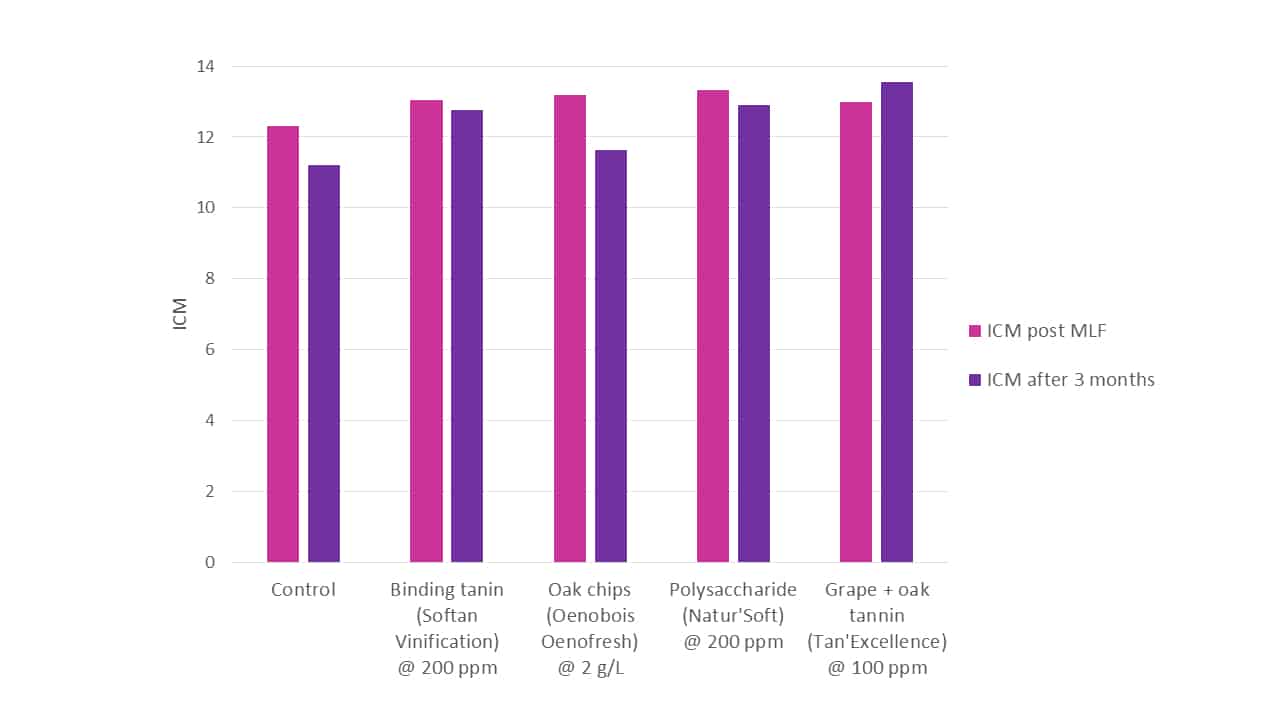
Figure 7. A comparison of total wine colour intensity (ICM) between various treatments measured post-MLF and again after 3 months. Treatments were added on day 2 of fermentation at levels recommended by the manufacturer, and were Softan Vinfication (colour-fixing tannin), Oenobois Oenofresh granular (oak chips), Natur’Soft (yeasty-derived polysaccharide; added day 3 of fermentation) and Tan’Excellence (grape + oak tannin).
So why not just add colour?
A viable question, and certainly an approach taken by some wineries when wine colour is deemed insufficient, but it is not without its problems. Additions of colour are not always stable, especially when made close to final packaging, which is perhaps no great problem if the wine is destined for rapid turnover. The underlying problem, however, is that pigmented materials are colloidal in nature, and changing the colloidal composition of a wine, especially late in the process and more so if a catalytic process such as cross-flow filtration is involved, can lead to significant packaging filterability problems (Bowyer & Edwards, 2014). Table 2 shows filterability data for one wine with added colour over 5 days, on receival, then 2 days later immediately after cross-flow filtration, then after 3 further days post-cross flow. Filterability index is seen to rise significantly 3 days after cross-flow filtration, due to the presence of the colouring additive and as a result of its colloidal impact on the wine in combination with the colloidal catalysis of the cross-flow process. The final filterability values are post-Beco 220 lenticular filtration (0.3-0.5 um nominal).
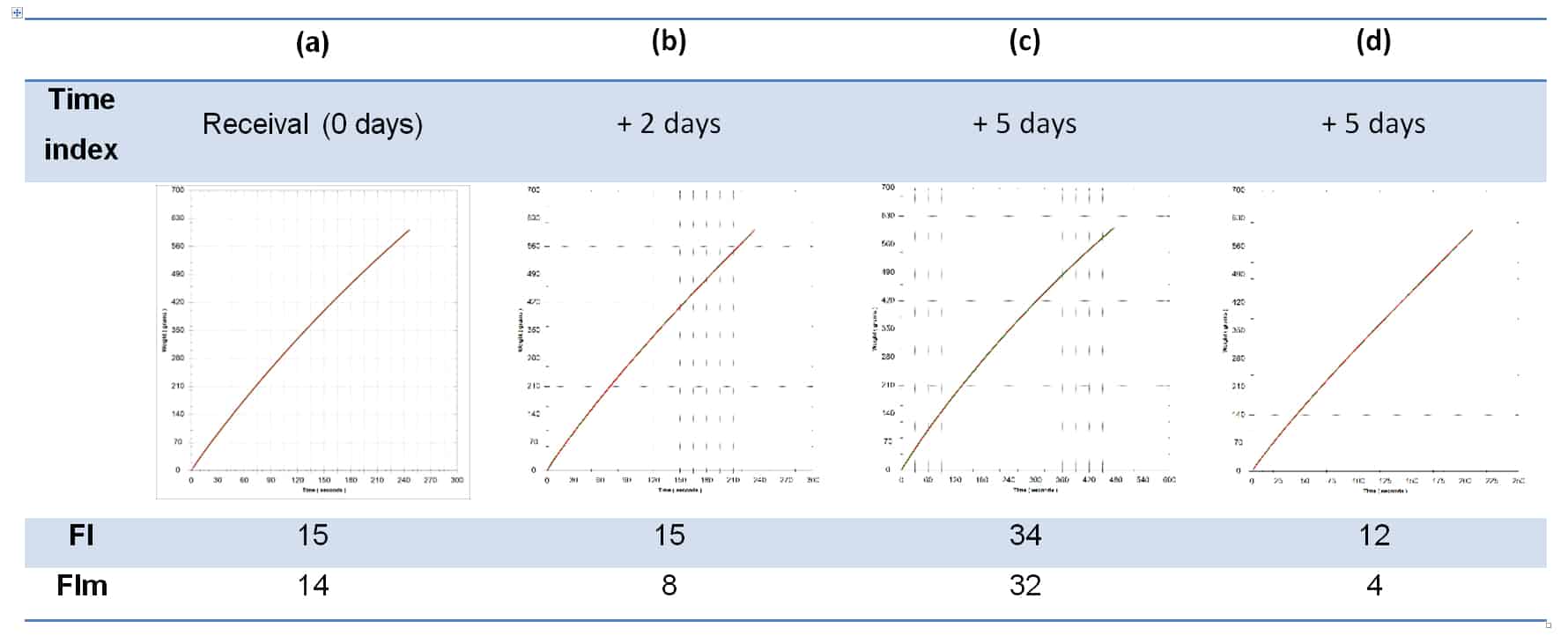
Table 2. Filterability data for the same wine at four time points after an addition of colouring material has been made. (a) The initial wine after colourant addition and post cross-flow on receival; (b) Immediately after a second pass through cross-flow 2 days after receival; (c) 3 days after the second cross-flow pass from (b); and (d) after passing wine from (c) through Beco 220 lenticulars (0.3-0.5 um).
Summary: A colour-fixing red winemaking protocol
Having discussed various ways in which a winemaker can seek to maximise and stabilise red wine colour, following are suggested winemaking considerations. This list is not prescriptive, but simply seeks to suggest an effective management strategy. Other factors should be considered too, such as coinoculation for malolactic fermentation (as this allows faster completion of MLF and hence earlier sulphite addition for better oxidation protection) and cold stabilisation alternatives (since traditional chilling often involves colour loss).
> Enzyme – use a purified, active enzyme, and allow it at least 4 hours to work after addition.
> Yeast – choose a yeast that generates a more tannic wine, and produces polysaccharide. Early coinoculation should be considered, which in turn requires an MLF-friendly yeast (Bowyer et al, 2017).
> Fining tannin – should be added early in the fermentation (day 1) once the enzyme has completed its action.
> Fixing tannin – should be added day 1 (end) or day 2 of fermentation, after the fining tannin has bound grape protein.
> Polysaccharide – add according to manufacturer’s instructions, but typically early in fermentation.
> Maturation tannin – consider using a maturation tannin to maintain the wine’s redox potential.
By Dr Paul Bowyer (Blue H2O Filtration) & Arnaud Mennesson (Lamothe-Abiet)
References
Bowyer, P. K. (2002) Phenolics: a peek inside the Pandora’s box of organic chemistry, The Australian and New Zealand Grapegrower and Winemaker, Annual Technical Issue, 67-70.
Bowyer, P. K. (2003) Molecular polarity – it’s behind more than you think, The Australian and New Zealand Grapegrower and Winemaker, November Issue, 89-91.
Bowyer, P. K. (2008) Proteinaceous fining agents, Part 1, The Australian and New Zealand Grapegrower and Winemaker, June Issue (533), 55-63.
Bowyer, P. K. (2009) Tannins vs oak chips: what does each contribute to your wine?, The Australian and New Zealand Grapegrower and Winemaker, April Issue (543), 61-65.
Bowyer, P. K. and Edwards, G. (2014) Understanding filterability index: an overview and some new insights The Australian and New Zealand Grapegrower and Winemaker, November issue (610), 80-85.
Bowyer, P. K. and McCord, J. (2007) StaVin – the alternative oak alternative, The Australian and New Zealand Grapegrower and Winemaker, July Issue, 73-78.
Bowyer, P. K., de Lumley, M. and Murat, M-L. (2010) A brief look at exogenous enzyme function in winemaking, The Australian and New Zealand Grapegrower and Winemaker, February issue (553), 43-50.
Bowyer, P. K., Chancholle, L. and Mennesson, A. (2017) Yeast breeding as a tool for wine stylistic manipulation, The Australian and New Zealand Grapegrower and Winemaker, October issue (645), 73-76.
Escot, S., Feuillat, M., Dulau, L., Charpentier, C. (2001) Release of polysaccharides by yeasts and the influence of released polysaccharides on colour stability and wine astringency, Australian Journal of Grape and Wine Research, (7), 153–159.
Gawel, R., Smith, P. and Waters, J. (2016) Influence of polysaccharides on the taste and mouthfeel of white wine, Australian Journal of Grape and Wine Research (22:3), 350-357.
Holt, H., Cozzolino, D., McCarthy, J., Abrahamse, C., Holt, S., Solomon, M., Smith, P., Chambers, P. J., and Curtin, C. (2013) Influence of yeast strain on Shiraz wine quality indicators, International Journal of Food Microbiology (165), 302-311.
Mercurio, M.D., Dambergs, R.G., Cozzolino, D., Herderich, M.J., Smith, P.A. (2010) Relationship between red wine grades and phenolics. 1. Tannin and total phenolics concentrations, Journal of Agricultural and Food Chemistry, (58) 12313–12319.
Morata, A., Gomez-Cordoves, M.C., Suberviola, J., Bartolome, B., Colomo, B., Suarez, J.A. (2003) Adsorption of anthocyanins by yeast cell walls during the fermentation of red wines. Journal of Agricultural and Food Chemistry (51) 4084–4088.
Ribéreau-Gayon, P., Glories, Y., Maujean, A. and Dubourdieu, D. (2004) Traité d’oenologie – Tome 2 – 5th ed., Chimie du vin. Stabilisation et traitements: Chimie du vin. Stabilisation et traitements (Traité d’oenologie (2)) (French Edition), 243.
BHF wishes to thank The Australian and New Zealand Grapegrower and Winemaker for permission to reproduce this article. Subscription information can be found here.